摘要:
目的:探讨海藻糖在动脉内皮细胞冻存过程中的保护作用。方法:通过在传统细胞冻存液中添加一定浓度的海藻糖对人主动脉内皮细胞进行液氮深低温冻存,根据cck-8结果将冻存液分为对照组(A组),50 mmol∙L
−1海藻糖组(B组)以及100 mmol∙L
−1海藻糖组(C组),运用台盼蓝染色法观察复苏后细胞的存活率,免疫印迹检测自噬标志性蛋白LC3B,Beclin-1、P62的表达情况,以及CCK-8检测细胞复苏后的增殖活性。结果:人主动脉内皮细胞经6个月液氮冻存后,细胞计数结果显示与A、C组相比较,B组活细胞比例明显增多(F = 7.50, P < 0.05);免疫印迹结果表明与A、C组相比,B组自噬标志性蛋白LC3B,beclin-1表达水平明显上升(F
LC3B = 36.88, F
beclin-1 = 135.6, P < 0.05),P62表达较对照组下降(F = 163.1, P< 0.05)。复苏后细胞增殖活性显示随时间的增加,细胞增值率逐步升高且B组增殖率较A、C组高(F
24 h = 14.14, F
48 h = 19.95, P < 0.05)。结论:低浓度海藻糖可在一定程度上有效提升冻存后细胞的活性,这种作用可能是通过促进自噬的发生而实现的。
Abstract:
Objective: To explore the protective effect of trehalose during the cryopreservation of arterial endothelial cells. Methods: Human aortic endothelial cells were cryopreserved in liquid nitrogen by adding a certain concentration of trehalose to the traditional cell cryopreservation solution. According to the results of CCK-8, the cryopreservation solution was divided into the control group (A), the 50 mmol/L trehalose group (B), and the 100 mmol/L trehalose group (C). Trypan blue staining method was used to observe the survival rate of resuscitated cells, and western-blot to detect the autophagy marker protein LC3B, Beclin-1 and P62 expression, and CCK-8 to test the cell proliferation activity after resuscitation. Results: After cryopreservation in liquid nitrogen for 6 months, the cell count showed that compared with group A and C, the proportion of viable cells in group B increased significantly (F = 7.05, P < 0.05); the result of western-blot showed that compared with A and C, the expression of autophagy marker proteins LC3B and Beclin-1 in group B increased (FLC3B = 36.88, Fbeclin-1 = 135.6, P < 0.05), and the expression of P62 decreased (F = 163.1, P < 0.05). After resuscitation, the cell proliferation activity showed an increase over time, and proliferation rate of group B was higher than the other groups (F24 h = 14.14, F48 h = 19.95, P < 0.05). Conclusion: Low-concentration trehalose can effectively enhance cell viability after cryopreservation, and this effect may be achieved by promoting the occurrence of autophagy.
1. 前言
同种瓣移植广泛应用于瓣膜病和先心病的外科治疗,但移植后的衰败钙化是影响其使用效果的主要原因。研究发现,移植前冻存过程中细胞活性的变化是影响移植后耐久性的因素之一 [1]。因此,如何提升冻存过程中组织细胞的活力是一个不可忽视的问题。二甲基亚砜(DMSO)是最常用于组织细胞冻存的渗透性冷冻保护剂,但其细胞毒性在冻存过程中会对细胞造成一定的影响 [2]。课题组前期研究发现,在冷冻保护剂中添加一定浓度的海藻糖有助于提升冻存后组织细胞的活性;此外,我们还通过在纳米颗粒的介导下将海藻糖导入细胞内,进一步提升冻存后细胞的活性 [3],但其具体机制仍然不清。有研究表明,海藻糖是一种自噬激活剂 [4]。因此,验证自噬海藻糖是否通过自噬途径在冻存过程中发挥一定的作用是本次实验的主要目的。
2. 材料和方法
2.1. 材料
细胞 人主动脉内皮细胞(HAECs)来源于青岛大学转化医学院。
试剂F12培养基购于西格玛奥德里奇(上海)贸易有限公司;胎牛血清购自上海依科赛生物制品有限公司;二甲基亚砜(DMSO)购自北京金克隆公司;胰蛋白酶购于大连美伦生物技术公司;海藻糖,RIPA蛋白裂解液购于北京Solarbio公司;LC 3B Antibody,Beclin-1Antibody;β-Actin Rabbit mAb购于美国Cell Signaling Technology公司;P62 Antibody购于AbMole奥默生物技术(上海)有限公司;Anti-rabbit IgG,HRP-linked Antibody购自上海爱必信生物公司;PageRuler™ Prestained Protein Ladder购于美国Thermo Scientific公司。
2.2. 实验方法
2.2.1. 细胞培养
将人主动脉内皮细胞(HAECs)置于含0.1胎牛血清的F12培养基中,于37℃、0.05 CO2培养箱中培养,期间在显微镜下观察细胞生长状态并进行换液,当细胞密度涨至80%~90%时进行传代和下一步实验。
2.2.2. CCK-8检测不同浓度海藻糖对细胞活力的影响
取对数生长期的主动脉内皮细胞,以适宜密度接种于96孔板,每孔100 μl,置于37℃、0.05 CO2培养箱中培养,待细胞完全贴壁后,每孔加入100 μl含有不同浓度海藻糖的F12培养基继续培养,24 h后吸出培养基,并用PBS清洗三次,每孔加入100 μl新鲜的F12培养基及10 μl CCK-8溶液,于37℃、0.05 CO2培养箱中继续培养1 h后,用酶标仪检测波长为450 nm处的吸光度,并计算细胞存活率(细胞存活率(%) = ((实验组OD值 − 空白组OD值)/(正常组OD值 − 空白组OD值)) × 100%)。
2.2.3. 细胞冻存
称取一定量的海藻糖溶于胎牛血清中,分别配置不同浓度的含海藻糖的血清冻存液,并用0.22 μm的滤器于超净台滤过。将消化后的细胞接种在六孔板,待细胞长满至六孔板面积的90%以上时,进行细胞冻存,冻存体系控制在1 × 106个/ml。冻存方案根据CCK-8结果分为三组:① control组(A组):FBS + 0.1 DMSO;② 50 mmol/L Tre组(B组):FBS + 0.1 DMSO + 50 mmol/L Tre;③ 100 mmol/L Tre组(C组):FBS + 0.1 DMSO + 100 mmol/L Tre。先将细胞放入程序性降温盒中放入−80℃冰箱保存24 h,随后转移至液氮中深低温冻存,经6个月冻存后取出细胞进行下一步检测。
2.2.4. 细胞复温后台盼蓝染色计数
将冻存后的细胞从液氮罐中取出,于37℃水浴锅中快速复苏,将冻存管中的细胞悬液转移至1.5 ml EP管中,1000 r离心5 min,弃上清,加入含有0.1胎牛血清的F12培养基重悬细胞,做成细胞悬液。取细胞悬液与0.004台盼蓝溶液按9:1混合后,取10 μl加入细胞计数板,进行显微镜下细胞计数。活细胞率(%) = 活细胞总数/(活细胞总数 + 死细胞总数) × 100 %。
2.2.5. 蛋白质印迹法检测自噬相关蛋白的表达
细胞复苏后,将冻存管中的细胞悬液转移至1.5 ml EP管中,1000 r离心5 min,弃上清,每管加入100 μl细胞裂解液置于冰上30 min,4℃,12000 r离心30 min,取上清,加入蛋白上样缓冲液,于金属浴上98℃煮沸10 min使其变性。继续进行聚丙烯酰胺凝胶电泳,转膜,封闭,一抗(LC3B, Beclin-1, β-actin) 4℃孵育过夜,相应二抗37℃孵育2 h,随后进行化学发光成像检测。
2.2.6. 冻存后的细胞增殖检测
细胞复苏后,离心,用含有0.1胎牛血清的F12培养基重悬细胞,每孔加入100 μl细胞悬液,放入37℃、0.05 CO2培养箱中分别培养12 h、24 h、48 h后,每孔加入10 μl CCK-8溶液,放入培养箱中继续培养1 h,检测OD 450 nm处的吸光度。计算每组不同时间段与12 h相比,细胞数的比例作为细胞增殖率。
2.3. 统计学分析
运用GraphPad Prism 8.0软件进行统计学分析,多组间的比较采用单因素方差分析(ANOVA),多组在不同时间点的比较采用析因设计的方差分析,结果以
± s表示,P < 0.05具有统计学意义。
3. 结果分析
3.1. 不同浓度海藻糖处理细胞后细胞活性
细胞经不同浓度海藻糖处理24 h后结果显示(见图1),随海藻糖浓度的升高,细胞活力逐渐降低,细胞活力依次为(97.98 ± 2.21)%、(94.42 ± 2.42)%、(79.66 ± 4.58)%、(54.47 ± 4.78)%、(2.66 ± 2.74)%;其中50 mmol/L海藻糖和100 mmol/L海藻糖组相比较,P > 0.05,两组间无明显差异。因此,我们将以50 mmol/L和100 mmol/L 海藻糖浓度分别作为实验组。
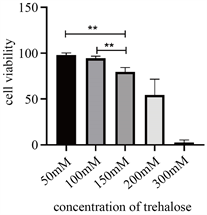
Figure 1. Cell viability after treated with different concentration of trehalose. (*P < 0.05, **P < 0.01)
图1. 不同浓度海藻糖处理后细胞活力(*P < 0.05,**P < 0.01)
3.2. 台盼蓝染色后计数
复苏后细胞计数结果显示(见图2) A、B、C三组的活细胞数占总细胞数的百分比分别为(92.90 ± 2.28)%、(96.75 ± 1.38)%、(92.49 ± 2.48)%,B组与A组及C组相比较,差异有显著性(F = 7.50, P < 0.05)。
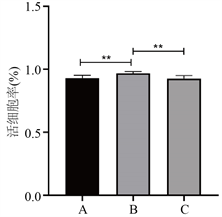
Figure 2. Proportion of living cells with trypan blue staining after cryopreservation. (*P < 0.05, **P < 0.01)
图2. 细胞复苏后台盼蓝染色活细胞比例(*P < 0.05,**P < 0.01)
3.3. 自噬相关蛋白的表达
免疫印迹结果显示(见图3),与对照组(A组)相比,B组及C组LC3II/I比值的相对表达量依次为 1.14 ± 0.01,1.05 ± 0.03,B组LC3B蛋白的表达量与A组及C组相比,差异有统计学意义(F = 36.88, P < 0.05);P62蛋白的相对表达量为0.86 ± 0.05,1.24 ± 0.02,差异有统计学意义(F = 163.1, P < 0.05);Beclin-1蛋白的相对表达量为1.08 ± 0.04,0.72 ± 0.05,差异有统计学意义(F = 135.6, P < 0.05)。结果表明细胞自噬的激活在此过程中发挥着一定的作用,且细胞自噬水平在50 mmol/L海藻糖组(B组)更为明显。
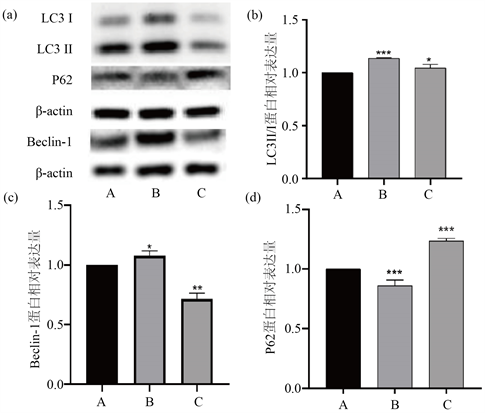
Figure 3. The expression of autophagy-related proteins. ((a) Western-blot picture of autophagy related protein; (b) The relative expression of LC3II/I; (c) The relative expression of Beclin-1 protein; (d) The relative expression of P62 protein)
图3. 细胞自噬相关蛋白的表达((a) 蛋白印迹图;(b) LC3II/I相对表达量;(c) Beclin-1蛋白相对表达量;(d) P62蛋白相对表达量)
3.4. 复苏后细胞功能评估
通过cck-8结果计算不同实验组不同时间段的细胞增殖率(见图4),单因素方差分析结果显示细胞培养后24、48 h细胞增值率逐步升高且B组增殖率较A、C组明显升高,差异有统计学意义(F24 h = 14.14, F48 h = 19.95, P < 0.05)。析因设计的方差结果显示(F组别 = 33.42, F时间 = 2973, F时间*组别 = 9.799, P < 0.05),表明不同冻存液浓度、培养时间以及其交互作用均对细胞增殖有一定的影响(见表1)。
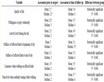
Table 1. The comparison of cell proliferation in different time points
表1. 各组细胞个时间点细胞增殖率比较(%)
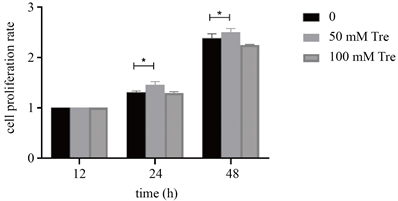
Figure 4. Cell proliferation after cryopreservation
图4. 细胞复苏后增殖率
4. 讨论
细胞自噬是一种自发降解胞内受损细胞器及错误折叠蛋白质的溶酶体降解途径 [5]。通过自噬,细胞可以将降解后所获得的氨基酸和能量再次代谢利用,从而保证了内环境的稳态。在正常生理条件下,自噬维持在一种较低的水平,且这种低水平的自噬是细胞生存所必需的 [6]。除此之外,当细胞受到一定信号的刺激时,如营养缺乏、缺氧、氧化应激等,将促进细胞自噬的发生,回收能量,从而在不利条件下维持细胞活力 [7] [8]。根据物质进入囊泡的机制,细胞自噬大致可以分为三大类,即巨自噬 [9],微自噬 [10] 及分子伴侣介导的自噬 [11]。
自噬标志性蛋白–微管相关蛋白1轻链3 (microtubule-associatedprotein1 lightchain 3, LC3)是一种参与自噬体成熟的受体蛋白 [12],它有LC3 I和LC3 II两种形式,其数量与自噬体形成的程度相关;SQSTM 1/p62是一种参与蛋白质聚集和连接多泛素化靶点的受体,两者都是自噬的标志基因。在动脉粥样硬化斑块与自噬的相关研究中发现,斑块早期的特点是高水平的LC3与SQSTM 1/p62共定位,表明早期自噬的发生以应对早期斑块的应激反应。而晚期病变的特征是LC3和SQSTM 1解离,表明该晚期中存在广泛的自噬功能障碍 [13]。Beclin-1又称BECN1,它不仅是自噬过程的另一个重要基因,在细胞凋亡中也发挥着一定的作用 [14]。现今的研究发现,自噬不仅存在于人体正常的生理代谢过程,它在疾病的发生发展中也扮演着重要的角色。人类的衰老 [15] 以及许多疾病,包括癌症 [16]、心血管疾病 [9]、神经退行性疾病 [17] 和免疫紊乱 [18] 等,都与细胞自噬具有相关性 [19]。
海藻糖是一种天然的非还原性二糖,广泛存在于如酵母、细菌、昆虫等低等生物中 [20]。当细胞暴露于如寒冷、炎热、干燥、氧化等环境变化时,海藻糖能作为一种典型的应激代谢物,以保护机体抵抗外部环境压力 [21] [22]。海藻糖是一种出色的生物保护剂,其特殊的结构和性质能够替代水分子中的氢键,稳定脂质、蛋白、病毒、血细胞,提高真核细胞应对失水环境的能力 [23]。有研究表明,低浓度细胞内海藻糖的引入可以大大提高冷冻保存过程中哺乳动物细胞的存活率 [24]。因其拥有无毒、高效的特点,海藻糖作为一种非渗透性冷冻保护剂被用于各种细胞、组织器官的冷冻保存 [25]。在细胞自噬方面,海藻糖是一种不依赖于雷帕霉素靶蛋白受体(mTOR)的自噬激活剂,如在神经系统疾病中,它可以通过促进自噬,从而加速亨廷顿疾病相关蛋白的清除,从而达到一定的治疗效果 [26]。在心血管系统中,有研究者发现海藻糖也能通过激活自噬减缓心肌梗死所致的心脏重塑以及心功能紊乱 [27]。综上所述,海藻糖介导的细胞自噬在许多疾病中都发挥着一定的作用。
5. 结论
本次研究,我们通过在传统DMSO冻存方案的基础上添加一定浓度海藻糖拟进一步提升经低温冻存后细胞的活性,研究结果显示低浓度海藻糖的存在一定程度上促进了细胞自噬的发生,且与对照组相比,海藻糖能对细胞发挥一定的保护作用。本次实验我们旨在初步探索海藻糖的低温保护作用与细胞自噬的相关性,有望为将来组织细胞冻存提供新靶点和新的思路。
基金项目
山东省科技发展计划项目(2013GHY11504)。
NOTES
*通讯作者。