1. 引言
哺乳动物大脑视交叉上核(SCN)由大约20,000个神经元组成,将生理和行为的昼夜节律与外界的近日节律同步 [1] [2] 。SCN神经元通过神经递质耦合达到同步,并输出一个统一周期。SCN可被导引至不同的外部光暗循环周期,此周期的范围称为导引范围,其中导引的最短/长周期称为导引下限(LLE)/导引上限(ULE)。不同物种的导引范围不同,例如人类的导引范围约为20.5至29小时,尼罗河小鼠的导引范围约为22.5至25.5小时 [3] 。
SCN神经元根据功能、释放的神经递质种类等可以分为两个子群:腹外侧区(VL)和背内侧区(DM),其中VL神经元约占总数的25%,接受外部传入的光信号,并释放VIP促进SCN生物钟神经元导引至外部周期,DM神经元释放的AVP被发现在持续条件(例如持续黑暗)下有利于SCN自振荡周期的稳定,VL和DM神经元均释放GABA [4] [5] [6] [7] 。已有研究表明,由于神经递质种类的差异,VL和DM神经元释放递质的强度并不完全相同 [8] [9] 。
两个子群的神经元在功能特性方面存在一定的异质性,例如周期、振幅等。研究证实VL神经元对光暗周期变化的再导引比DM神经元更快 [4] 。最近,新的神经元特性弛豫速率被发现,它代表了振子对外部振幅扰动的刚性 [10] [11] 。SCN神经元的弛豫速率有所不同,变化范围在0.03至1 h−1 [1] 。有文献研究了不同弛豫速率条件下,SCN网络的导引能力 [12] 。但目前为止,这种差异与神经元网络的耦合异质性对SCN导引功能的影响尚未得到充分研究。
本文建立了改进的Poincaré模型,假设VL神经元接受外部光信号,研究当SCN的两个子群VL和DM的弛豫速率存在异质性时,它们释放神经递质的强度,即平均场耦合的贡献如何影响LLE,并给出相应的理论解释。
2. 模型介绍
本文使用Poincaré模型用于模拟SCN网络暴露在外部光暗循环导引能力。Poincaré模型包含神经元振子的振幅和相位,每个神经元振子由两个变量x和y描述,由一个平均场F实现全局耦合 [1] [13] [14] [15] 。由N个神经元组成的SCN网络动力学方程如下:
(1)
其中
分别为第i个振子弛豫速率、固有振幅和固有周期。振子通过平均场耦合,在方程中表示为
,g为耦合强度,平均场F的定义为:
(2)
其中,
为振子在平均场的权重,在本文中,
和
分别表示振子i属于VL和DM子群,两个子群的
分别定义为:
(3)
其中,引入
来表征
的异质性,当
,所有神经元对SCN网络的作用相等,平均场F无
异质性;当
,VL振子释放神经递质的强度大于DM振子,对平均场的贡献更大;当
,DM振子对平均场的贡献大于VL振子。为了描述弛豫速率的异质性,本文假设
和
,令
作为两个子群弛豫速率的比值。因为VL神经元作为接受并向其他神经元传递光信号的主体,因此假设
和
。
当SCN神经元在接受外部信号时的周期
与外部周期T满足公式:
,其中
时,我们认为SCN成功被导引至外部周期。导引范围可以用导引下限(LLE)表示,即可被导引的最短周期。LLE越大,导引范围越小,反之亦然。本文中的LLE定义为:
,其中
为自由运行周期。
其他参数根据参考文献 [1] 确定:
。数值模拟采用四阶龙格–库塔方法,时间增量为0.01 h。为了避免瞬态的影响,忽略了最初的2000,000个时间步长(20,000 h)。网络大小设置为
。每个变量的初始条件从
的均匀分布中随机选择。
3. 结果与分析
3.1. 数值模拟结果
当
和
时,SCN网络对22小时外部光暗循环的导引结果由图1所示。由图1(a),当
时,VL振子的弛豫速率更小,
时SCN网络未能导引至22小时的外部周期。随着
的增大,当
时,SCN网络能够导引至22小时周期;但当
进一步增大至
时,SCN的导引能力减弱,未能导引至22小时周期。另一方面,当
时,
及
的情况下SCN都未能导引至22小时的外部光暗周期,当
时,SCN将内部周期成功导引至22小时。此结果说明了不同的平均场异质性所带来的导引能力差异随两个子群弛豫速率的比值而变化。
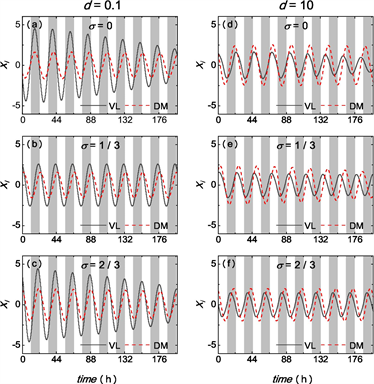
Figure 1. Time series of the impact of different
on SCN for
and
under an external period of 22 hours
图1. 外部周期22小时下
和
时不同
对SCN导引影响差异的时间序列图
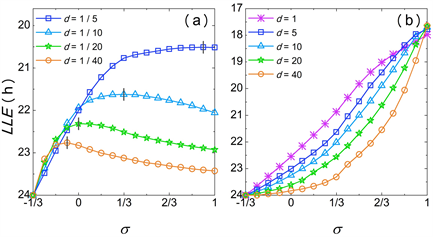
Figure 2. Diagram of the entrainment range of SCN under different values of d with different
图2. 不同d下
对SCN导引范围的分析图
在不同d的条件下,
对SCN导引范围的影响由图2所示。导引下限的时间轴为逆序(从24小时周期开始减少)以表征导引范围的扩大。由图2(a)可得,当
时,SCN的导引范围随
的增大先增大,并在
时趋于20.5小时;当
时,SCN的导引范围随
的增大先增大后减小。由灰色标记可得,最大值分别出现在
时,呈递减顺序。由图2(b)得,当
时,导引范围随
的增大单调递增。两种不同的变化趋势分别出现在
及
,说明
对导引范围的影响会随着弛豫速率比值d的变化而变化。
图3展示了不同
的条件下,d对SCN的导引范围影响差异。首先,当
时,导引范围随着d的增大先增大后减小,且导引范围的最大值不断增大;当
时,导引范围先增大后接近于18小时。从图3可以看出,在
的条件下,导引范围的最大值并不出现在
,即完全由VL振子提供耦合作用。而在
的条件下,当
时导引范围要远大于其他
值下的导引。
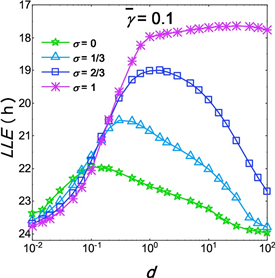
Figure 3. Diagram of the entrainment range of SCN under different values of
with different d
图3. 不同
下d对SCN导引范围的分析图
3.2. 解析结果
用解析结果来分析数值实验的合理性,令
,其中振子a表示VL神经元,振子b表示DM神经元,平均场
。方程(1)表示为:
(4)
为了化简,将方程转化为极坐标,其中
,得
(5)
本文采用由Krylov和Bogoliubov提出、文献 [13] [14] [15] [16] 中使用的平均化简方法,令
,有
(6)
其中
表示对时间求平均,将上式代入方程(5)可得
(7)
由上式可得,为得到LLE (
),
应近似于−1,则
近似于0。取得LLE时两振子的相位差
,因此
,由方程(7)得
(8)
首先,考虑
的情形,此时
,且随着
的增大,
递增,
递减,由方程(8)的第2和4行可知,
均单调递增。其次,考虑
的情形,此时
,当
时,随着
的增大,
递增,
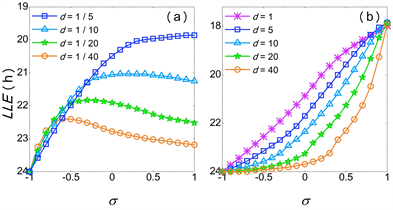
Figure 4. Diagram of the entrainment range of SCN under different values of d with different
for
图4.
时不同d下
对SCN导引范围的分析图
递减,
单调递增。随着
,有
,因此
取决于较小值。又因为随着
,
递增,
递减,所以数值实验中的导引范围先增大后减小。图4展示了
时在不同d的条件下
对SCN导引下限(LLE)的影响,其中两个振子分别表示VL和DM,与图2的结果较为相似。
4. 研究结论
本文对SCN神经元释放递质和弛豫速率的异质关系建模,并研究在VL和DM神经元的弛豫速率不同的条件下,神经递质释放的异质性对SCN的导引范围(以导引下限LLE为度量标准)产生的不同影响。数值实验表明:
1) 当VL与DM的弛豫速率比值
时,SCN的导引范围随着
(VL神经元释放神经递质强度)的增大先增大后减小。弛豫速率比值d越小,达到导引范围峰值的
值越小。
2) 当VL与DM的弛豫速率比值
时,SCN的导引范围随着
的增大单调增加。当VL神经元对平均场的贡献度最大时,SCN网络具有最大导引能力。
由于SCN两个子群神经元释放的神经递质存在差异,未来的实验可以通过测量VL神经元和DM神经元的递质释放强度和弛豫速率,更好地解释SCN神经元网络的导引机制和不同物种导引范围的差异。
基金项目
国家自然科学基金(12275179和11875042)与上海市自然科学基金(21ZR1443900)。
NOTES
*通讯作者。