1. 引言
诺贝尔奖得主费曼于1959年美国物理学会年会上所作经典演讲中 [1] ,提出了纳米科技的概念,并预测人们将在纳米尺度上制备并控制物质的性质。分子机器属于纳米科技范畴,是迄今为止最小尺度的微纳机电系统,它通过自下而上的化学合成和自组装等方法加以实现 [2] ,并通过将能量转变为分子构件的可控运动实现某些功能。虽然分子机器的研究还远没达到可以成熟应用的阶段,但由于它具有小尺寸、多样性、自适应,以及仅依靠热能、光照或者化学能驱动等其他人造机器难以比拟的许多优点,因而在生物医药、新材料、传感器以及储能系统的研发中具有广阔的潜在应用前景,因而它的发展具有重要意义 [3] 。1983年,索维奇等人成功地将两个环形分子连接起来,形成一根链,并将其命名为“索烃” [4] ,其中一个环能在接受能量后受控绕另一个环旋转,这是分子机器的雏形。1991年,斯图达特等人利用分子间的互相吸引,研究出“轮烷”,他将一个“轮烷”分子环穿入一个细的分子轴,并通过加热时,使环随轴产生前窜后跳运动 [5] 。之后,斯图达特和他的团队以“轮烷”为研究基础,2004年构建分子电梯 [6] ,2005年构建人造肌肉 [7] 。1999年,费林加等人研究出分子转动叶片,成功地使叶片持续朝一个方向旋转,并保持此方向不变 [8] ,2011年又实现了反方向旋转 [9] 。在2011年,费林加研究组还制造了一个四轮驱动的“纳米车” [10] 。由于在分子机器研究方面的杰出贡献,索维奇、斯图达特和费林加共同获得 2016年的诺贝尔奖。
热能驱动是分子机器常用的一种驱动方式,而热膨胀性质是材料在受热时呈现的一种重要的物理性质,研究环状分子这种分子机器最常见的构件的热膨胀性质,显得十分重要,然而在目前还未见有关环状分子热膨胀性质理论和实验研究方面的报道。由于分子机器尺寸过于微小,目前对其构件的热膨胀性质进行实验研究还比较困难,因此我们将在本文中对环状分子的热膨胀性质进行理论研究。分子动力学方法常被运用于材料的热膨胀性质模拟,由于这是一种纯粹的基于牛顿力学的数值计算方法,因此不能通过解析公式分析物理机制,又由于忽略了量子效应因而结果也不准确,所以本文将不采用分子动力学方法。晶格动力学方法曾经被我们成功地运用于晶体薄膜热膨胀性质的理论研究 [11] [12] ,本文也将借助于晶格动力学理论推导环状分子热膨胀性质的计算公式并进行数值计算,最后分析其热膨胀性质。
2. 环状分子的振动
设有
个质量为
的相同原子构成的环状分子,最近邻原子平衡位置间隔为
,相互间的线性力常数为
。由于环状分子长度
等于
,故可用
描述环状分子长度。设原子
的平衡位置沿环方向的坐标为
为
。由于只有沿环的纵波才能引起热膨胀,因此只需考虑环状分子中的纵波。根据晶格动力学,原子
的振动位移可表示为 [13] :
(1)
其中,
为原子质量,
和
是声子产生与消灭算符。波矢
和角频率
之间符合以下色散关系:
(2)
环状分子振动哈密顿可表示为
(3)
其中,
是声子数算符,平均声子数符合玻色统计:
(4)
3. 环状分子的热膨胀公式
将原子间相互作用势进行泰勒级数展开,热膨胀由其非和谐项引起,
阶非和谐项可表示为:
(5)
和谐晶体中不存在热膨胀。在和谐晶体中引入非和谐势能项后,由于热振动导致非和谐晶体晶格常数相对于和谐晶体晶格常数有一个变化量,该变化量称为晶格常数热膨胀。根据微扰论,我们曾得非和谐势能
引起晶格常数热膨胀公式 [11] [12]
(6)
其中,
或
分别是由和谐近似下各种
的声子态的直积构成的未微扰态。
根据原子位移公式及产生与消灭算符的性质可知,上式中的
和
状态相差一个声子,设该声子波矢为
,可以将(6)式中的
和
分别表示为
(7)
(8)
将(5)、(7)和(8)式代入(6)式,得原子间距热膨胀
(9)
其中
(10)
将(10)式代入(9)式,得
(11)
根据(1)式,不难发现,只有奇数阶的非和谐势能项对热膨胀才有贡献。设
,则
(12)
将(1)代入上式,得
(13)
根据热膨胀系数定义,
阶非和谐势能项引起的热膨胀系数可表示为
(14)
其中,
和
分别为和谐近似下平均到单个原子的振动内能和振动比热
。
可由下式计算
(15)
4. 数值计算结果与分析
虽然氩原子构成的环形构件在分子机器中没有具体应用,但由于氩原子间的相互作用能用L-J模型 [14] 准确描述,因此我们通过对它进行计算来了解环状分子的热膨胀性质的尺寸效应。
长度N为6和80个氩原子的环状分子的原子间距热膨胀随温度变化如图1所示,而其热膨胀系数随温度的变化如图2所示。由图1可知,在0 K温度下原子存在零点振动,因而在0 K温度下环状分子中原子间距热膨胀不为零。由图1和图2可知,环状分子原子间距热膨胀以及热膨胀系数都随温度增加而增加,短环状分子的热膨胀小于长环状分子的热膨胀,短环状分子的热膨胀系数大于长环状分子的热膨胀系数,并且在低温情况下这些差异非常明显,而当温度升高时差异明显减弱。因此,只有在低温下才有必要考虑环状分子热膨胀性质的尺寸效应。
在0.1 K温度下,环状分子的原子间距热膨胀随长度N的变化如图3所示,而热膨胀系数随长度N的变化如图4所示。由图3和图4可知,只有在环状分子较短时才有必要考虑其热膨胀性质的尺寸效应。当环状分子的长度较短时,随着环状分子的长度增加,热膨胀有显著增加,而热膨胀系数有显著减小。而当环状分子的长度较长时,随着环状分子的长度增加,热膨胀系数不再有明显减小,而热膨胀不再有明显增加,它们都各自趋于一个极限值,由(13)和(14)式知,这些极限值与无限长环状分子的比内能、比热有关,还取决于环境温度。
5. 结论
本文运用晶格动力学理论和微扰理论,推导出环状分子的热膨胀及热膨胀系数公式,在此基础上进行了数值计算,最终根据数值计算结果总结环状分子的热膨胀性质的尺寸效应规律。研究结果表明,在低温下,并且环状分子较短时,环状分子热膨胀性质具有明显的尺寸效应,即随着环状分子长度的增加其原子间距热膨胀有显著增加,而同时环状分子的热膨胀系数有显著减小。随着温度的升高,或当环状
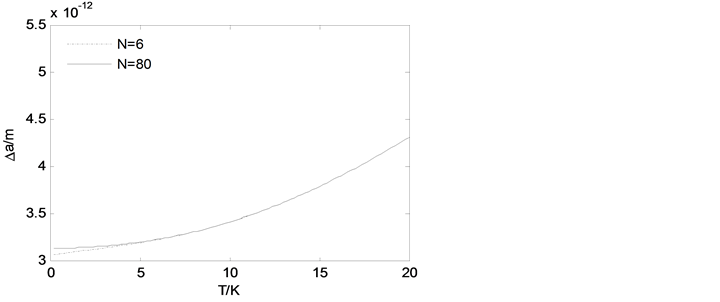
Figure 1. Thermal expansion of interatomic distance in cyclic molecule vs. temperature
图1. 环状分子中原子间距热膨胀与温度关系
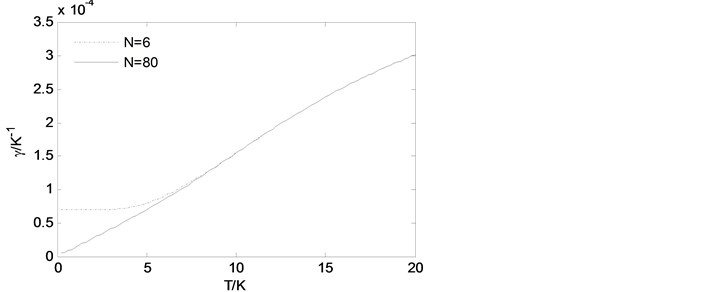
Figure 2. Thermal expansion coefficient of cyclic molecule vs. temperature
图2. 环状分子热膨胀系数与温度关系
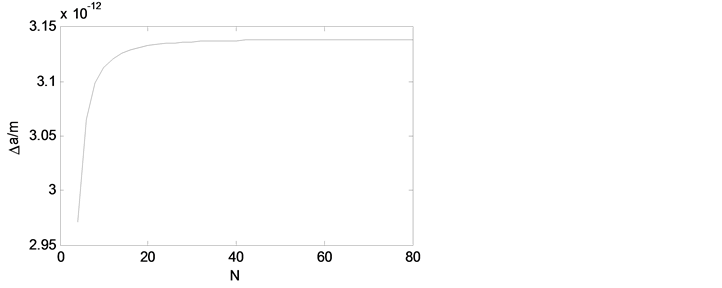
Figure 3. Thermal expansion of interatomic distance in cyclic molecule vs. its length
图3. 环状分子中原子间距热膨胀与分子长度关系
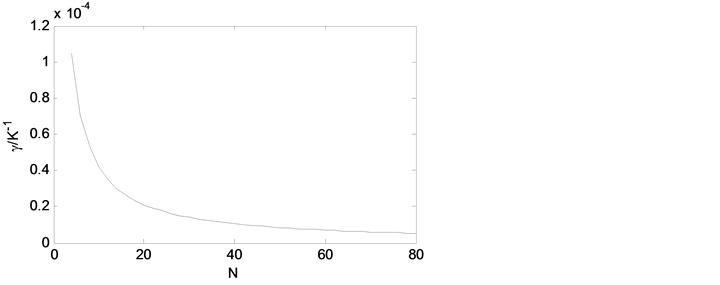
Figure 4. Thermal expansion coefficient of cyclic molecule vs. its length
图4. 环状分子热膨胀系数与分子长度关系
分子长度较长时,环状分子热膨胀性质的尺寸效应明显减弱直至消失,即随着环状分子长度的增加其原子间距热膨胀不再有显著增加,同时环状分子的热膨胀系数也不再有显著减小。因此在分子机器中,必须考虑在低温下的短环状分子的热膨胀性质的尺寸效应。
NOTES
*通讯作者。