1. 引言
非酒精性脂肪肝已经成为世界上最常见的慢性肝病之一,据统计目前全球患病率为25.24%,且全球各地区的患病率呈上升趋势,大大增加了社会的经济负担 [1],但目前尚未有良好的治疗方式,主要以饮食和运动来控制 [2] [3]。非酒精性脂肪性肝病 (Nonalcoholic fatty liver disease, NAFLD)包括从单纯性脂肪变性到非酒精性脂肪性肝炎和肝硬化的一系列肝脏表型 [4]。虽然大多数NAFLD患者仅具有无进展的脂肪变性,但相当一部分会发展为非酒精性脂肪性肝炎(Nonalcoholic steatohepatitis, NASH),并进一步进展为肝硬化、肝细胞癌(Hepatocellular carcinoma, HCC),导致肝脏相关死亡率增加 [5]。
有研究证明肠道微生物区系与代谢相关性疾病(非酒精性脂肪肝、肥胖和糖尿病)的发病密切相关 [6] [7],肠道的代谢产物可以通过门静脉循环进入肝脏,影响一些受体或者炎症细胞来介导肝脏的氧化应激以及炎症免疫反应 [8]。但高脂饮食介导的非酒精性脂肪肝模型小鼠的肠道菌群及代谢产物的变化是不固定的,所以其对疾病的进展尚未有明确定论。本研究我们通过给小鼠喂食高脂饲料构建非酒精性脂肪肝模型,利用16S rDNA微生物测序技术和非靶向代谢组学分析肠道菌群及代谢产物的变化,探索其对非酒精性脂肪肝的影响,为非酒精性脂肪肝发病机制以及诊断提供一定的基础。
2. 方法与材料
2.1. 小鼠饲养与处理
购买自济南朋悦实验动物繁育有限公司的30只SPF级5周龄雄性C57/BL6J小鼠适应性喂养7天,后将小鼠随机分为2个小组,包括非酒精性脂肪肝模型组(High-fat Diet, HFD组)以及对照组(Control check,CK组),小鼠饲养在18℃~24℃的室温环境中,光暗对照各12小时,每天自由饮水及进食,饲养16周。所有动物操作流程均按照动物实验室伦理准则进行。
2.2. 组织收集
喂食16周后使用代谢笼收集小鼠粪便,液氮速冻15 min后转入−80℃冰箱用于后续分析。然后小鼠禁饮食12 h后采血处死,解剖小鼠收集肝脏,部分保存于4%多聚甲醛,用于肝脏H.E染色及油红染色,剩余保存于−80℃冰箱用于后续分析。血液经离心机(2000 rmp, 15 min)离心后采集上清液用于血液生化分析。
2.3. DNA提取及16S rRNA基因测序
采用DNA抽提试剂盒(QIAGEN,12888)对粪便菌群DNA进行提取,之后利用琼脂糖凝胶电泳和NanoDrop2000 检测DNA的浓度。用针对V3-V4区域的特异引物343F (5'-TACGGRAGGCAGCAG-3')和798R (5'-AGGGTATCTAATCCT-3')扩增细菌DNA,检测质量并纯化。纯化后作为二轮PCR模板进行扩增,后PCR产物进行Qubit定量。将等量的纯化扩增物汇集到Illumina MiSeq平台进行后续测序。测序数据进行预处理后,采用Vsearch软件,根据序列的相似性,将序列归为多个可操作分类单元(OTU)。使用QIIME软件包挑选出各个OTU的代表序列,通过RDP classifier将所有代表序列与Silva (version123)数据库比对注释,保留置信区间大于0.7的注释结果。
2.4. 粪便代谢组学分析
粪便经过预处理后使用Nexera UPLC超高效液相串联QE高分辨质谱仪组成的液质联用系统,分析了在ESI正离子和负离子模式下的代谢谱。采用ACQUITY UPLC HSS T3(1.8µm, 2.1 mm × 100 mm)进行正、负模式实验。二元梯度洗脱体系由A-水(含0.1%甲酸)和B-乙腈(含0.1%甲酸)组成,流速0.35 mL/min。初始组成为95% A和5% B,采用以下梯度进行分离:95%A,0.01~2 min;70%~95% A,2~4 min;50%~70% A,4~8 min;20%~50% A,8~10 min;0~20% A,10~14 min,在100% B条件下保持2 min; 0-95% A,15~15.1 min,95% A,15.1~16 min。在整个分析运行过程中,QC样品以固定间隔(每10个样品)注入,以提供一组可用于评估重复性的数据。采集的LC-MS原始数据通过progenesis QI v2.3软件进行分析。
2.5. 统计学分析
血生化指标采用SPSS 26.0软件进行非参数检验,用Wilcoxon秩和检验比较两个对照组间α多样性的差异,并利用加权Unirac距离矩阵数据进行主坐标分析(PCOA)以可视化β多样性。组间的LEfSe分析利用Kruskal-Wallis和秩检验进行了显著性检验,组间的两两比较采用Wilcoxon秩和检验对其进行了生物学显著性检验。利用线性判别分析(LDA)获得最终的区分种。用PICRUST从16S rRNA序列中推测肠道菌群的元基因组,根据Wilcoxon算法对预测的KEGG数据库结果进行计数。多变量统计分析使用正交偏最小二乘幂分析(OPLS-DA)来区分组间代谢谱的整体差异,并找到代谢产物在群体之间的不同,应用了默认的7轮交叉验证,在每轮中将七分之一的样本排除在数学模型之外,以防止过度拟合。差异代谢物的筛选标准为OPLS-DA模型第一主成分为VIP > 1,t检验P < 0.05。利用KEGG数据库对差异代谢物进行通路富集分析。此外,我们利用超几何检验来识别与整体背景相比,在显著差异表达的代谢物中显著富集的通路条目,认为P< 0.05的通路显著富集。采用Spearman相关检验(Student’s t检验,P < 0.05,|相关系数| > 0.3)分析肠道菌群与肠道代谢产物的相关性。所有的分析和图形使用QIIME(1.8.0)、R软件(3.6.2)和R软件(3.5.1)进行。所有数值均以平均值±SD表示。P < 0.05认为结果有统计学意义。
3. 结果
3.1. 肝脏的病理改变及血生化指标
经过16周干预后,小鼠肝脏切片HFD组与CK组比较肝脏出现明显脂肪变性(图1)。血生化指标(表1)显示,小鼠HFD组ALT,AST及甘油三酯明显升高。
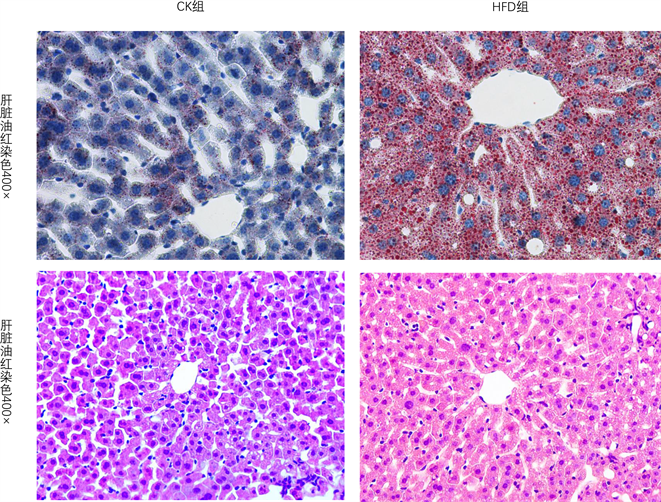
Figure 1. Liver steatosis oil red staining was observed in the high fat diet group. Blue staining for nucleus, red staining for lipid droplets; H.E staining of liver, red staining of cytoplasm and blue staining of nucleus. 400× is the magnification of tissue under the microscope
图1. 高脂饮食组肝脏出现明显脂肪变性油红染色,蓝染表示细胞核,红染代表脂滴;肝脏H.E染色,胞质红染,胞核蓝染。400×为组织在显微镜下的放大倍数
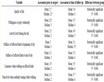
Table 1. Comparison of blood biochemical analysis between the two groups (n = 15 per group,
)
表1. 两组血液生化分析比较(n = 15只/组,
)
3.2. 低聚木糖对小鼠肠道菌群的影响
α多样性Chao1指数明显降低表明HFD组中肠道菌群丰度明显减少(图2(a))。PCoA分析可以观察到两组间距离明显(图2(b)),同时Adonis分析P < 0.001,表明肠道菌群分布及结构存在显著差异。
通过Wilcoxon分析两组间不同水平的肠道菌落差异,主要展示了门、种和属水平的菌群差异。在门水平,CK组中放线菌及梭杆菌丰度升高(图2(c),图2(d));在属水平中,HFD组与CK组共有46种差异菌群,16种菌落在CK组丰度升高,30种降低,下图展示了属水平的前10种差异肠道菌群,7种菌群丰度在CK组中升高(图2(e),图2(f));在种水平,共有19种差异菌群,其中10种在CK组种升高,图中展示了种水平前10种差异菌群,其中有6种在CK组种升高(图2(g),图2(h))。
通过LEfSe分析进一步确定了NAFLD模型小鼠的特定菌落和生物标志物,LEfSe分析共发现了15个鉴别特征(P < 0.05, LDA > 4),在门水平,CK组放线菌丰度水平显著增加;在纲水平,CK组Coriobacteriia,Bacilli丰度升高;在目水平,CK组小鼠Coriobacteriales,Lactobacillales丰度升高;在科水平,Atopobiaceae,Lactobacillaceae在CK组丰度升高,Prevotellaceae,Rs_E47_termite_group丰度在HFD组中升高,在属水平,HFD组中Faecalibaculum,Alloprevotella,uncultured_bacterium丰度升高,A2,Lactobacillus及Coriobacteriaceae_UCG_002在CK组中丰度升高(图3(a))。
基于16S rDNA数据,通过PICRUSt分析预测了小鼠肠道菌群功能组成。两组共有179个不同的KEGG通路,下图显示了差异最显著的前50项。结果显示,许多KEGG通路包括鞘脂代谢,甘油磷脂代谢,脂肪细胞因子信号通路,PPAR信号通路等可能与NAFLD的发生机制有关(图3(b))。后续基于LCMS的
(a)
(b)
Figure 3. Results of LEfSE analysis and PICRUSt analysis. (a) T-axis family tree plots obtained by LEfSe analysis and Wilcoxon test of 16S sequence using linear discriminant analysis (LDA) effect size (LEfSe) analysis. LEfSe identified the groups with the greatest differences in abundance between CK and HFD groups at phylum, class, order, family and genus levels. HFD group is red, CK group is green, and only LDA threshold reaches > 3; (b) PICRUSt analysis results for predicting functional pathways of intestinal flora
图3. LEfSE分析和PICRUSt分析结果。(a) 利用线性判别分析(LDA)效应大小(LEfSe)分析和16S序列的Wilcoxon检验获得的T轴系谱系图。LEfSe鉴定出在门、纲、目、科和属水平上CK组和HFD组丰度差异最大的类群。HFD组表示为红色,CK组为绿色,仅显示LDA阈值达到 > 3的菌群;(b) 预测肠道菌群功能通路的PICRUSt分析结果
代谢组学分析也支持了一些通路。
3.3. 非靶向组学分析肠道差异代谢产物
我们采用正交偏最小二乘方–判别分析(Orthogonal Partial Least Squares-Discriminant Analysis, OPLS-DA)表征两组之间的整体代谢组学差异。结果显示,各组样本在OPLS-DA评分图中均具有明显的分离。这说明,在高脂饮食干预的小鼠粪便中,代谢产物存在显著差异(图4(a))。
为防止模型过拟合,采用七次循环交互验证(7-fold cross validation)和200次响应排序检验(response permutation testing, RPT)的方法来考察模型的质量。下图表明OPLS-DA模型具有良好的稳定性,不存在过拟合问题(图4(b),R2 = 0.87, Q2 = −0.65)。
然后通过OPLS-DA评分和t检验,两组共鉴定出371种显著变化的差异物(VIP > 1, P < 0.05)。371种代谢产物进一步分类,包括脂质和脂样分子(180),生物碱及其衍生物(4),类苯(8),木酚素、新木酚素及相关化合物(1),核苷、核苷酸和类似物(2),有机酸及其衍生物(34),有机氮化合物(1),有机氧化合物(11),有机单环化合物(15),苯基丙酮类化合物和聚酮类化合物(13),未分类代谢物(102)。与对照组比较,HFD组中有156种代谢产物降低,在这些代谢物中,主要为脂肪酸及共轭物、氨基酸、多肽和类似物等,下图主要展示了两组间前50的差异代谢物(图4(c))。
我们对差异代谢产物进行了KEGG功能富集分析。图中显示了具有统计学意义的代谢途径(P < 0.05) (图5(a), 图5(b)),有研究证实甘油磷脂代谢,鞘脂代谢,细胞衰老都可能与非酒精性脂肪肝的发病有关 [9] [10]。在甘油磷脂代谢途径中,HFD组小鼠肠道中甘油磷脂、2-溶血磷脂酰胆碱平均含量降低,磷脂酸水平升高,在鞘脂代谢途径中,鞘氨醇、鞘氨醇1磷酸在HFD组小鼠中水平升高。
3.4. 肠道菌群与差异代谢产物之间的联系
我们通过Spearman分析研究了属水平差异肠道菌群与前50的代谢产物之间的联系,如图所示,我
(a)
(b)
(c)
Figure 4. Changes of intestinal metabolites in NAFLD mice. (a) OPLS-DA score charts of CK group and HFD group; (b) Response sequencing test to verify whether the model is over-fitting; (c) The expression levels of the metabolites with significant differences and the 50 differentially expressed metabolites with the highest VIP values were analyzed by hierarchical clustering (VIP > 1, p < 0.05)
图4. NAFLD小鼠肠道代谢产物的变化。(A) CK组与HFD组的OPLS-DA评分图;(b) 响应排序检验,用于验证模型是否过拟合;(c) 通过层次聚类分析存在显著差异的代谢物以及VIP值最高的50个差异表达代谢物的表达水平(VIP > 1, p < 0.05)
(a)
(b)
(c)
Figure 5. KEGG analysis of differential metabolites. ((a), (b)) Metabolic pathways with the highest concentration of differential metabolites; (c) Comparison of levels of differential metabolites in metabolic pathways. P < 0.05, indicating statistically significant difference
图5. 差异代谢物KEGG分析。((a), (b)) 差异代谢物富集程度最高的的代谢途径;(c) 代谢通路中差异代谢产物的水平比较。P < 0.05,代表差异有统计学意义
们重点关注了鞘氨醇,甘油磷酸胆碱等物质。甘油磷酸胆碱与Coriobacteriaceae_UCG-002,Lactobacillus成正相关,与Prevotella_7成负相关。鞘氨醇与Lachnospiraceae_NK4A136_group,Coriobacteriaceae_UCG-002,Tyzzerella,A2,GCA-900066225,Ruminococcus_1,Simplicispira,Family_XIII_UCG-001等肠道菌群成负相关,与Hymenobacter,Corynebacterium_1呈正相关(图6)。
4. 讨论
非酒精性脂肪性肝病已经是世界上最常见的慢性肝病之一,且肠肝轴被认为是非酒精性脂肪肝发病中的重要参与者,多项研究表明肠道菌群紊乱可以通过门静脉循环促进非酒精性脂肪肝的疾病进展,如加剧炎症反应等 [6] [11] [12]。在本次研究中,我们在HFD组中磷脂酸高表达,甘油磷酸胆碱、2-溶血磷脂酰胆碱低表达,通过KEGG通路甘油磷脂代谢可以得知LPIN、磷脂酶A2等基因参与磷酸酯,甘油磷酸胆碱的代谢。通过与肠道菌群的关联分析,我们发现甘油磷酸胆碱与Coriobacteriaceae_UCG-002,Lactobacillus成正相关,与Prevotella_7呈负相关。甘油磷酸胆碱(GPC)是一种类似于磷脂酰胆碱的胆碱前体,已有证据表明GPC具有一定的促进脂质代谢的功能,可以减少脂质的局部累积 [13]。另外,Coriobacteriaceae和Lactobacillus均在CK组中升高,有研究表明Coriobacteriaceae可以减轻胰岛素抵抗 [14],并可改善肝脏脂肪变性 [15]。同时乳酸杆菌也被证明可以通过降低胆固醇及肝脏脂肪变性来改善
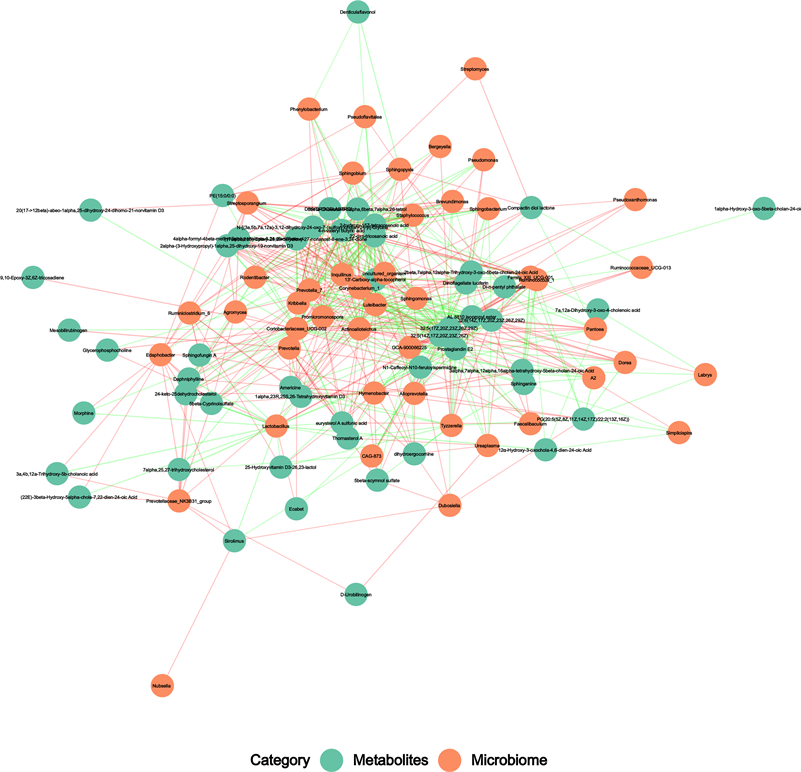
Figure 6. Potential correlations between gut microbiota and metabolites. Correlation between genus level intestinal bacteria with the first 50 metabolites, the red line represents positive correlation, the green line represents negative correlation, and the thickness of the line represents correlation coefficient.
图6. 肠道微生物群与代谢物之间的潜在相关性。属水平肠道细菌属与前50种代谢产物的相关性,红线表示正相关,绿线表示负相关,线的粗细表示相关系数。
NAFLD的进展 [16]。而Prevotella_7被报道是一种与高膳食纤维有关的一种菌群,可以有效改善脂肪变性及炎症 [7],且在CK组中低表达,这与甘油磷酸胆碱的功能一致,所以我们推测这些菌群可能通过干预甘油磷酸代谢途径中的LPIN、磷脂酶A2等基因的表达来影响NAFLD的进展,其中的机制需要进一步研究。
鞘氨醇是一种鞘磷脂,是鞘氨醇1-磷酸的底物,它可以被鞘氨醇激酶-1 (SphK1)磷酸化为鞘氨醇1-磷酸(S1P)。有证据表明,鞘氨醇1-磷酸及鞘氨醇激酶是肝脏脂质积累和脂肪组织中脂质储存的重要参与者 [17],它们可以通过激活S1P-Akt-mTOR信号通路调节PPAR-γ来影响肝脏的脂肪变性及炎症 [18]。但也有证据显示鞘氨醇激酶-2 (SphK2)过表达可以增加S1P,减少体内鞘氨醇及神经酰胺的含量,并且可以减轻肝脏的胆固醇及甘油三脂的积累,减轻胰岛素抵抗 [19]。通过我们的研究显示,鞘氨醇在CK组中低表达,且与Coriobacteriaceae_UCG-002,Ruminococcus_1,Tyzzerella,A2等细菌成负相关,Ruminococcus_1被证明是一种可以产生丁酸的菌群,丁酸可以调节非酒精性脂肪肝的脂肪变性 [20]。上文也提出Coriobacteriaceae_UCG-002可以改善非酒精性脂肪肝的脂肪变性,这表明Coriobacteriaceae_UCG-002,Ruminococcus_1可能也具有通过影响鞘脂代谢途径来改善脂肪变性的功能,可以降低鞘氨醇激酶1或者使鞘氨醇激酶2过表达,来降低体内鞘氨醇的含量,减少S1P的产生,减少肝脏细胞的脂肪变性,其中的机制需要进一步明确。
5. 总结
非酒精性脂肪肝会引起肠道菌群的改变,不进行干预,肠道菌群就无法恢复,这可能是非酒精性脂肪肝发生发展的重要因素。另外,我们通过LEfSE分析得出了非酒精性脂肪肝不同门类水平的生物标志物,并对差异微生物功能进行了预测,为进一步从菌群角度对NAFLD进行干预提供了重要参考。鞘氨醇以及鞘氨醇1磷酸增加可能会促进非酒精性脂肪肝的进展,而甘油磷脂可能会改善NAFLD的进展,后续有必要增加样本量,并进行药物干预,进一步了解非酒精性脂肪肝的发病机制。
NOTES
*通讯作者Email: 17691341791@163.com