1. 引言
多孔材料由于其较大的比表面积和开放式骨架结构赋予的优异催化、吸附等性能,被广泛应用在各类催化剂、吸附剂领域 [1] 。90年代,Gier T. E.团队合成得到具有优异性能的磷酸锌晶体,使得金属磷酸盐这一类物质走进研究人员的视野。随着多样化的金属元素和不同的骨架结构被引入,相比于金属磷酸盐,过渡金属磷酸盐具有更开放的结构,更多元化的金属氧化态,使其展现出良好的质子传导性能 [2] [3] 。然而,电子导电性抑制了过渡金属磷酸盐的催化性能,有研究表明将导电材料与过渡金属磷酸盐结合能在一定程度上实现协同催化,从而进一步拓宽过渡金属磷酸盐的应用前景 [4] [5] 。相比于常见的过渡金属磷酸盐制备方法,如水热合成法、溶剂热合成法以及固相合成法等 [6] ,微波辅助加热合成法具有反应时间短、速率快等优势成为研究热点。
最新研究表明,前列腺癌在男性癌症中的死亡率和发病率有逐步上升的趋势。2009年,sreekumar团队 [7] 发现人体内肌氨酸水平与前列腺癌具有一定的相关性,相比于常见的直肠指检和前列腺特异性抗原筛查,肌氨酸检测作为一个无创选项在前列腺癌早筛中具有应用意义。和传统的液相色谱法 [8] 、气相色谱法 [9] 和荧光法 [10] 等相比,电化学法 [11] [12] 具有便捷且低成本的优势,但是也存在抗干扰能力不佳的劣势。在前期的研究工作中,Wang等人发现通过改变表面电荷密度可以间接地提高传感器的抗干扰能力 [13] ,相较于传统的抗干扰手段如涂覆Nafion膜或者Chitosan膜,电化学沉积得到的导电聚合物薄膜不仅能自由控制薄膜厚度,提高修饰器件的导电性能,还能提高检测灵敏度 [14] [15] 。常见的导电聚合物单体如3,4-乙烯二氧噻吩(EDOT) [16] ,吲哚及其衍生物 [17] 等,单一条件下的沉积会导致其聚合物存在一定的局限性,然而在一定比例下的共沉积却可以弥补单一聚合物产生的缺陷 [18] ,有利于改善共聚物薄膜的性能。
受上述研究启发,本文以5'-三磷酸腺苷(ATP)为磷源,通过微波辅助加热合成法合成玫瑰花状的磷酸锰(Manganese Phosphate, MP),并将其与导电聚合物复合后电化学沉积成传感器薄膜,在此基础上制备出电流型肌氨酸传感器。将EDOT单体,吲哚-6-羧酸(IA)单体和MP混合后,使用电化学沉积的方法将其均匀的沉积在玻碳电极(Glassy Carbon Electrode, GCE)上,随后涂覆上肌氨酸氧化酶(SOx),即可制成一个简易的电流型肌氨酸传感器。这项工作为过渡金属磷酸盐的合成制备建立一套新方法,也为电流型传感器检测低浓度待测物质提供了一个新的抗干扰思路。
2. 实验部分
2.1. 试剂与仪器
试剂:四水合氯化锰(分析纯)、四氟硼酸四丁基铵(分析纯)、ATP (分析纯)、EDOT (分析纯)、IA (分析纯)和无水乙腈(分析纯)均购自上海阿拉丁生物有限公司;Sox (分析纯)购自J & K scientific Ltd;肌氨酸(分析纯,Sarcosine)、抗坏血酸(分析纯,Ascorbic acid,AA)和尿酸(分析纯,Uric acid,UA)购于国药集团化学试剂有限公司。
仪器:透射电镜(Tecnai G2 F20)和XPS (Microlab 350),Thermo Fisher Scientific;微波辅助加热合成仪(XH-300UL),Beijing Xianghu Science and Technology Development Co., Ltd;扫描电镜(Hitachi U70),Hitachi Limited,Japan;电化学工作站(CHI660C),Shanghai Chenhua Instruments。
2.2. MP的控制合成
MP的合成:分别用30 mL去离子水溶解MnCl2∙4H2O (0.198 g)和ATP (0.11 g),然后将ATP滴加进MnCl2水溶液中,随后将溶液pH调节至5 (0.1 M NaOH)。将混合液转移到微波合成反应釜中,微波反应加热到120℃ (加热速率5℃∙min−1,保温10 min),离心干燥,即可得到MP。
2.3. 沉积母液的制备
沉积母液的制备:四氟硼酸四丁基铵(0.1 M)、IA (0.02 M)、EDOT (0.1 M)和MP (2 mg∙mL−1)溶解在10 mL的乙腈溶液中,混合均匀即可配置成沉积母液。
2.4. 传感器的制备
传感器的制备:将打磨抛光好的GCE浸没到沉积母液中,通过电化学沉积(扫速50 mV∙s−1,沉积电压窗口−0.8~1.2 V,沉积圈数4圈)得到修饰电极,标记为PEDOT-IA/MP/GCE。将SOx (10 μL, 4 U∙μL−1)用戊二醛溶液(0.1 M)固定在PEDOT-IA/MP/GCE,即可得到肌氨酸传感器,标记为SOx/PEDOT-IA/MP/GCE。
3. 结果与讨论
3.1. MP的表征
图1(a)是MP的SEM图片,从图中可以看出微波水热合成的MP呈纳米片堆砌而成的玫瑰花状,纳米片之间有空隙存在,使得大量催化活性位点暴露出来。图1(b)是MP的TEM图片,MP微观形态呈玫瑰花状,与图1(a)相互印证。图2(a)和图2(b)分别是MP和O1s的XPS图谱,从O1s在528~534 eV处的图谱中可以发现化合物结构中有Mn-O-P组分 [19] 的存在,说明MP被成功的合成出来。
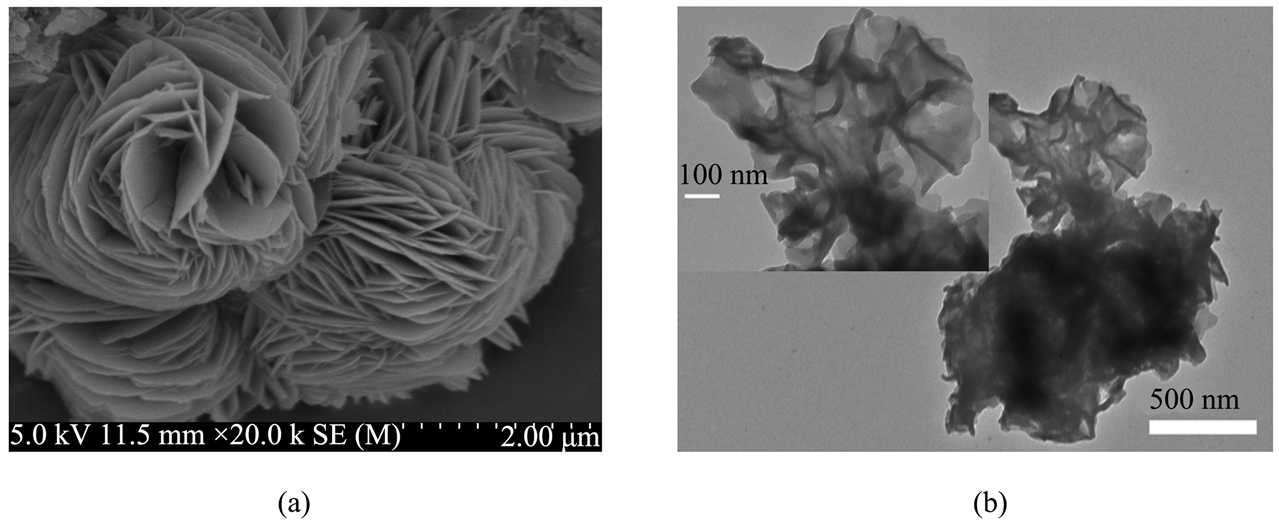
Figure 1. SEM image (a) and TEM image (b) of MP
图1. MP的SEM图片(a)和TEM照片(b)
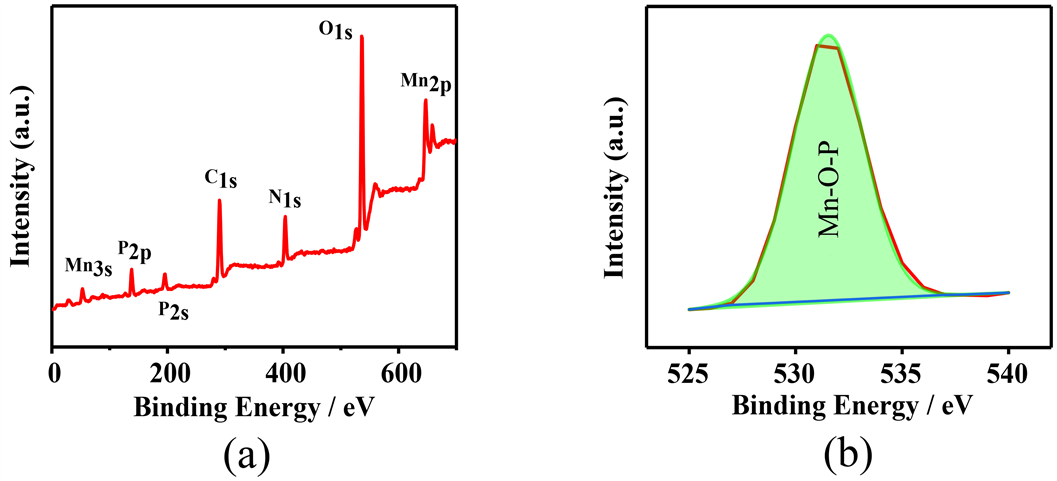
Figure 2. (a) XPS spectrum of MP; (a) XPS spectrum of O1s
图2. (a) MP的XPS光谱;(b) O1s的XPS光谱
3.2. MP的制备条件优化
将不同参数下微波合成的MP滴涂在GCE上,以其对1 mM过氧化氢(H2O2)的氧化电流值作为参考,制备参数的优化如图3所示。随着pH值逐渐趋近中性值时,H2O2电流值呈现一个先增后减的趋势,归因于ATP在低pH值的溶液中的水解被抑制,当pH值为5时达到最佳pH条件,如图3(a)所示。图3(b)所示为反应时间对MP合成过程的中影响,在10 min时所测的H2O2电流值最大,后续增加反应时间电流值变化不明显,这可能是由于合成时间过长导致MP的纳米花结构完整性受到破坏,催化活性位点暴露减少引起的;当反应温度为120℃时,对应的电流值达到最大,如图3(c)所示,这是温度过高会加快ATP的水解。因此,当pH为5时,120℃反应10 min可制备最优性能的MP。
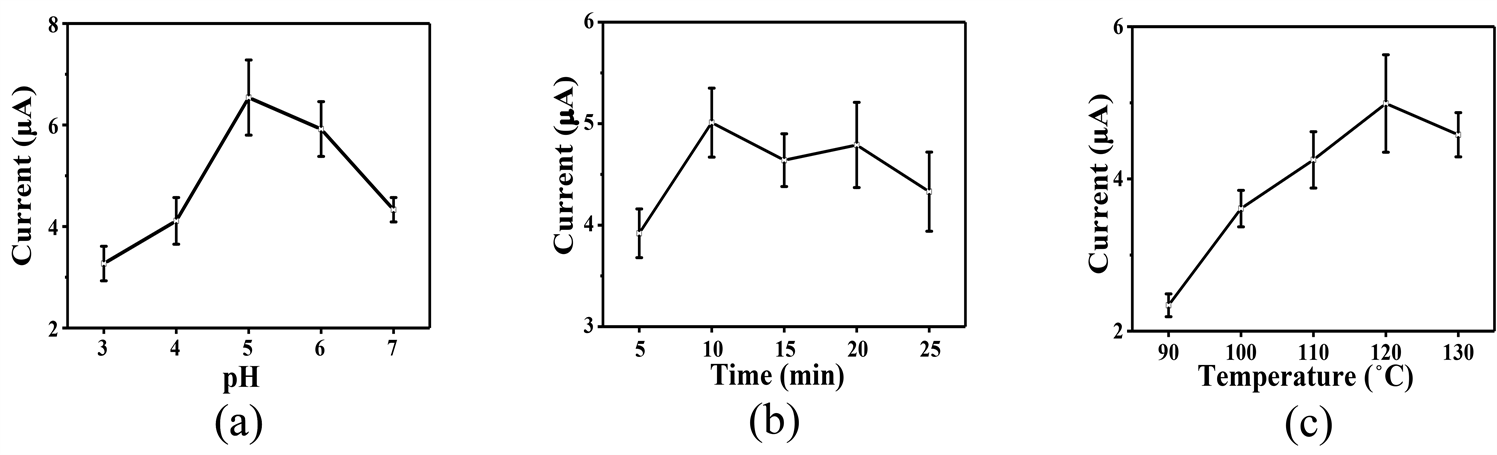
Figure 3. Optimization of MP preparation conditions: (a) pH; (b) Reaction time; (c) Reaction temperature
图3. MP的制备条件优化:(a) pH;(b) 反应时间;(c) 反应温度
3.3. PEDOT-IA/MP/GCE制备条件优化
将不同含量的MP分散在沉积母液中,通过电化学沉积的方式优化PEDOT-IA/MP/GCE的制备方式,如图4所示。图4(a)为沉积圈数的优化,可以看到随着沉积圈数的增加,在第4圈时PEDOT-IA/MP/GCE对10 μM AA的响应值最低,进一步增加沉积圈数,响应值不降反升。这归因于沉积圈数的增加导致PEDOT-IA/MP/GCE表面电负性增加,对于抗坏血酸的排斥增加,响应信号下降。MP的用量如图4(b)所示,在2 mg∙mL−1时PEDOT-IA/MP/GCE对1 mM H2O2的电流值达到最大,增加MP用量时,电流值呈现下降趋势,这可能是过多的MP导致沉积层过厚,稳定性变差。因此,在含有2 mg∙mL−1的MP母液中沉积4圈,可获得最佳电化学性能的PEDOT-IA/MP/GCE。
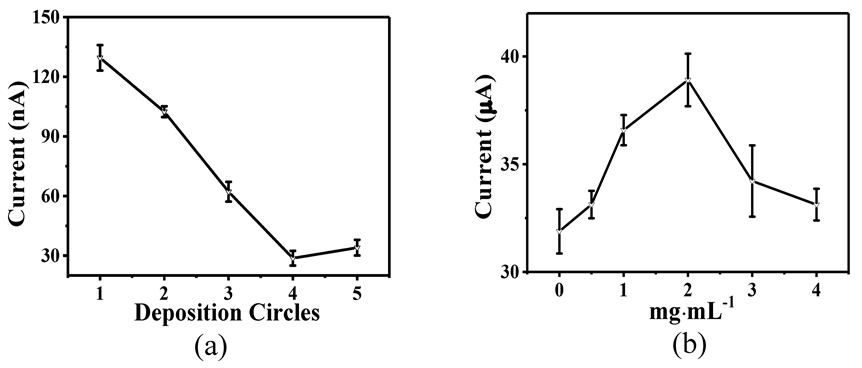
Figure 4. Optimization of preparation conditions for PEDOT-IA/MP/GCE: (a) number of deposition circles; (b) MP concentration
图4. PEDOT-IA/MP/GCE制备条件的优化:(a) 沉积圈数;(b) MP的用量
3.4. SOx/PEDOT-IA/MP/GCE的电化学性能测试
从图5(a)可以看出,相比于单独的MP/GCE和PEDOT-IA/GCE,PEDOT-IA/MP/GCE在铁氰化钾溶液中的CV曲线结合了MP/GCE和PEDOT-IA/GCE的优势,展现出良好的氧化还原性能和导电性能,说明PEDOT-IA与MP共沉积后得到具有优异电化学性能的PEDOT-IA/MP/GCE。EIS图可以间接用来表征传感器的制备,如图5(b)所示,相比于Bare GCE,分别制备的PEDOT-IA/GCE、PEDOT-IA/MP/GCE和SOx/PEDOT-IA/MP/GCE展现出来的电化学阻抗性能不同,也间接说明了SOx/PEDOT-IA/MP/GCE被成功制备出来。SOx/PEDOT-IA/MP/GCE对不同浓度的肌氨酸呈现良好的响应性能,如图5(c)和图5(d)所示。SOx/PEDOT-IA/MP/GCE的线性检测范围为1~50 μM,拟合方程为I (μA) = 9.88C (μA∙mM−1) + 0.14195。估算得到传感器灵敏度为9.88 μA∙mM−1,检出限为0.16 μM (S/N = 3)。
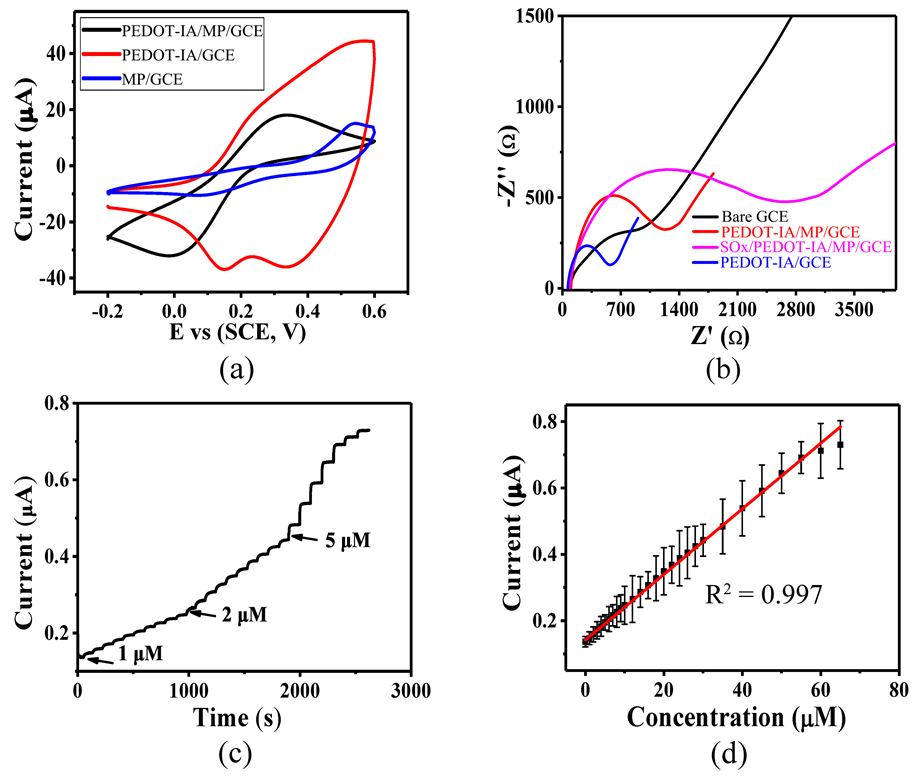
Figure 5. (a) CV curves of MP/GCE, PEDOT-IA/GCE and PEDOT-IA/MP/GCE (containing 5 mM potassium ferricyanide of 0.1 M KCl at a sweep speed of 50 mV∙s−1); (b) EIS curves from Bare GCE, PEDOT-IA/MP/GCE, SOx/PEDOT-IA/MP/GCE and PEDOT-IA/GCE; (c) I-T curves of SOx/PEDOT-IA/MP/GCE for different concentrations of sarcosine; (d) The relationship between the corresponding concentration and the response current
图5. (a) MP/GCE,PEDOT-IA/GCE和PEDOT-IA/MP/GCE的CV曲线(含0.1 M KCl的5 mM铁氰化钾,扫速50 mV∙s−1);(b) Bare GCE,PEDOT-IA/MP/GCE,SOx/PEDOT-IA/MP/GCE和PEDOT-IA/GCE的EIS曲线;(c) SOx/PEDOT-IA/MP/GCE对不同浓度肌氨酸的I-T曲线;(d) 对应的浓度与响应电流关系
3.5. SOx/PEDOT-IA/MP/GCE的抗干扰性能测试
如图6所示,在同样的干扰物浓度下(100 mΜAA和UA),SOx/PEDOT-IA/MP/GCE相比于Nafion和Chitosan膜响应电流值分别降低了71%和85% (以AA为例),说明SOx/PEDOT-IA/MP/GCE相比于传统电化学传感器抗干扰用的Nafion和Chitosan膜,在同样的干扰物浓度下具有更优异的抗干扰性能。这归因于SOx/PEDOT-IA/MP/GCE对同样带负电荷的AA和UA有着相互排斥的作用,减少干扰物质在传感器表面发生化学反应,从而使得SOx/PEDOT-IA/MP/GCE展现出来优异的抗干扰性能。
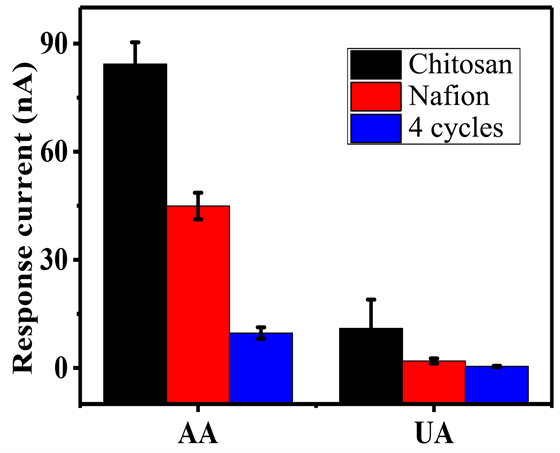
Figure 6. Anti-interference performance of SOx/PEDOT-IA/MP/GCE
图6. SOx/PEDOT-IA/MP/GCE的抗干扰性能
4. 结论
综上所述,通过微波辅助加热合成法合成玫瑰花状的MP与EDOT、IA混合后,采用电化学沉积的方法可以制备得到具有优异性能的传感器。当溶液pH为5时,120℃下微波反应10 min可制备出最优性能的MP,在含有2 mg∙mL−1的MP的沉积母液中电化学沉积4圈,可获得最佳电化学性能的敏感薄膜,并在此基础上制备的肌氨酸传感器具有良好的抗干扰性能,其线性检测范围为1~50 μM,灵敏度为9.88 μA∙mM−1,检出限为0.16 μM (S/N = 3)。
参考文献