1. 引言
以氟利昂为主的传统气体制冷技术,对于社会生态环境造成了巨大破坏。而以磁卡效应为原理的新型功能材料,可以有效的解决该问题。磁卡效应(MCE)是通过施加外磁场来改变磁性材料磁矩的有序度,进而引起材料磁熵的变化,最后导致材料的吸放热。其特征通常是在外加磁场强度发生改变时产生等温磁熵变化(ΔS)和绝热温度变化(ΔTad),同时这两种变化在居里温度TC附近改变最快 [1]。
R-M (R为稀土元素,M为3d过渡族元素)金属化合物因温度或磁场变化而引起多相转变,正不断吸引着基础科学和应用领域研究人员的兴趣 [2] [3] [4] [5] [6]。Laves相化合物是在已知的R-M化合物中最多的。许多RM2化合物在低温下会发生结构相变,值得注意的是过渡金属Co与不同稀土形成的化合物相变类型不同,例如:当稀土为Dy,Ho和Er时为一级相变,其他镧系元素时为二级相变 [7] [8]。因此,RCo2(R = 稀土)化合物的磁性机理一直被深入研究 [9] [10]。众所周知,相较于一级相变,二级相变具有低滞后、宽温域等优势。而之前的研究表明,对ErCo2掺杂过渡族元素Fe可以实现一级相变到二级相变的改变 [11]。因此本文通过过渡族元素Ni掺杂DyCo2实现:DyCo2的一级相变到Dy(Co0.5Ni0.5)2的二级相变的改变,以及Dy(Co0.5Ni0.5)2的高制冷能力。
2. 实验方法
首先利用Dy(Co0.5Ni0.5)2的化学元素比来进行配料,其中Dy的纯度为99.9%,Co和Ni的纯度为99.99%。由于稀土金属Dy在熔炼过程中容易挥发,因此,在配料过程中将Dy过量5%以补偿挥发所造成的损失,防止其它杂质相的形成。将原料放置在氩气氛围的电弧熔炼炉中反复熔炼五次,使合金充分熔化混合保证其均匀性,最后得到Dy(Co0.5Ni0.5)2铸锭。随后将Dy(Co0.5Ni0.5)2铸锭密封在石英管中,并将其放入箱式炉中在900℃的温度下退火5天。将退火后的样品使用综合物性测量系统(PPMS)测量样品的磁化曲线。以2 K/min的速率将样品冷却至100 K,由于样品为块体,在达到指定温度时,样品内部温度可能并未达到指定温度。因此,在达到指定温度后维持该温度20 min左右再进行测量,避免因样品温度引起的实验误差。在样品温度稳定之后以30 Oe/s的速率增加磁场并记录数据。随后再以4 K的温度间隔降温,在达到指定温度后维持该温度20 min之后再增加磁场进行测量,测量的温度区间为100 K至44 K。最后将测量出的磁化曲线数据使用Matlab进行数据处理,计算出Dy(Co0.5Ni0.5)2的磁熵变值。
3. 结果与讨论
图1是不同温度下(44 K到100 K之间)测量的等温磁化曲线,温度间隔为4 K,图中数据均为增加磁场时所测量出来的数据。从图1中能够看出在同一温度下,随着磁场的增加磁矩值不断增加。
通过Dy(Co0.5Ni0.5)2的等温磁化曲线可以绘制出Arrot曲线,结果如图2(a)所示。根据A. Banerjee判据,Arrott曲线的斜率可以用来判断相变的类型:如果曲线的斜率表现为负则是一级相变;如果曲线的斜率表现为正则是二级相变 [12] [13]。图中Arrot曲线的斜率均为正,也就说明了Dy(Co0.5Ni0.5)2的相变类型是二级相变。
根据Gibbs自由能和系统处在能量最小的状态时
,可以得出:
(1)
所以系数A(T)、B(T)和C(T)可以用H和M的多项式拟合来获得。A(T)和B(T)的计算结果如图2(b)所示。根据Inoue-Shimizu模型,A(T)为反转磁化率,会在TC处达到最小值。而在TC处的B(T)决定着样品的相变类型:对于一级相变B(T)为负值,对于二级相变B(T)为非负值 [14] [15] [16]。从图2(b)中可以看出,当T = TC时B(T)为正,这说明了Dy(Co0.5Ni0.5)2的相变为二级相变。
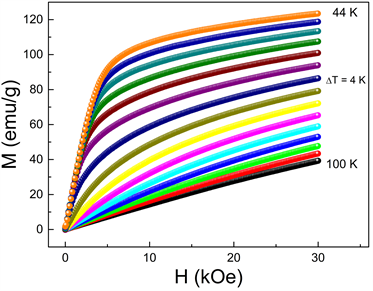
Figure 1. Magnetic field dependence of the magnetization for Dy(Co0.5Ni0.5)2
图1. Dy(Co0.5Ni0.5)2的等温磁化曲线
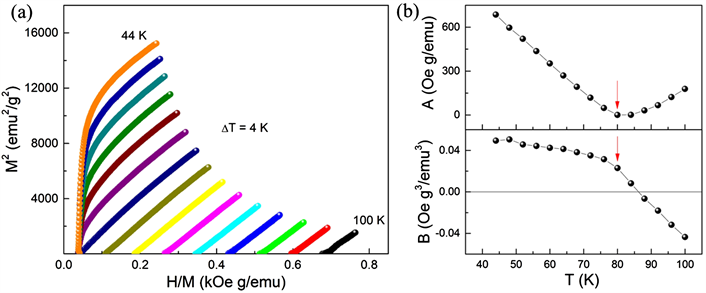
Figure 2. (a) Arrott plots of Dy(Co0.5Ni0.5)2 and (b) The fitted parameter A(T) and B(T) as a function of temperature of Dy(Co0.5Ni0.5)2
图2. (a) Dy(Co0.5Ni0.5)2的Arrott曲线和(b) Dy(Co0.5Ni0.5)2的A(T)和B(T)与温度的关系图
根据热力学第二定律以及热力学的麦克斯韦关系式可以推导出磁熵变ΔS与温度T、外加磁场H产生的磁化强度M之间的关系:
(2)
在本文中我们主要利用分离磁场下温度与磁化强度的关系曲线计算磁熵变,所以ΔS可以近似由以下方程得到 [17]:
(3)
通过等温磁化曲线和公式(3)我们能够得到在不同外加磁场下-ΔS随温度变化的曲线,如图3所示。从图中可以看到在30 kOe的磁场中Dy(Co0.5Ni0.5)2的磁熵变是最大的,其最大值为6.86 J/kg∙K。
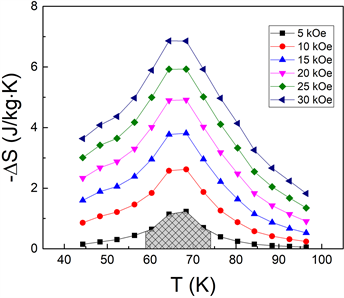
Figure 3. Magnetic entropy change vs temperature of Dy(Co0.5Ni0.5)2 at different magnetic field
图3. Dy(Co0.5Ni0.5)2在不同外加磁场下的熵变随温度变化关系示意图
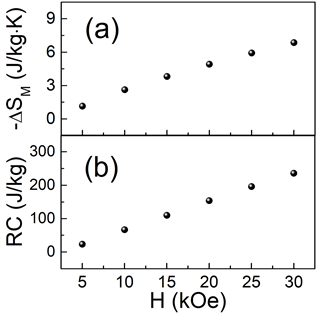
Figure 4. (a) Maximum magnetic entropy change and (b) Refrigerant capacity vs magnetic field of Dy(Co0.5Ni0.5)2
图4. Dy(Co0.5Ni0.5)2的(a)最大磁熵变(-ΔSM)和(b)制冷系数(RC)与磁场的关系图
除了磁熵变,制冷系数(RC)同样也是磁卡效应中的重要性质。制冷系数(RC)为
(4)
式中
和
为
时的温度。RC即为曲线和横轴所围成的面积,如图3中阴影部分所示。图4(a)和图4(b)分别是最大磁熵变(ΔSM)和RC随外加磁场变化的关系图。
通过文献调研,我们得到了其它Laves相合金在10 kOe下的制冷系数 [18] [19] [20]。由图5可知,Dy(Co0.5Ni0.5)2的制冷系数是这些合金中最高的,它远远大于TC较高的其它合金,略高于TC较低的合金,这使它有着更好的应用前景。
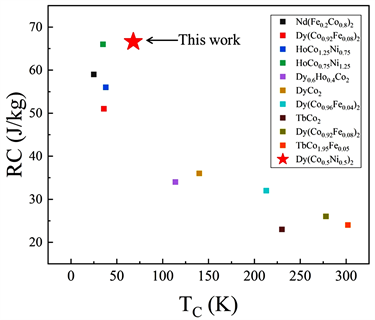
Figure 5. Refrigeration coefficients of different Laves phase compounds
图5. 不同Laves相化合物的制冷系数
4. 结论
采用电弧熔炼和真空热处理的方法制备了Dy(Co0.5Ni0.5)2合金,测量了Dy(Co0.5Ni0.5)2合金的等温磁化曲线。依据Arrott曲线的斜率为正以及在TC处B(T)的值为正确定了Dy(Co0.5Ni0.5)2合金的相变类型为二级相变。通过等温磁化曲线计算出了Dy(Co0.5Ni0.5)2的磁熵变,并且在30 kOe的磁场中其磁熵变最大值为6.86 J/kg∙K,制冷系数为235.78 J/kg。与其它Laves相合金对比可以看出在10 kOe的磁场中,Dy(Co0.5Ni0.5)2的制冷系数高于其它合金,这使它有着较好的应用前景。
基金项目
本研究受到了国家自然科学基金No.11475086项目的资助。
NOTES
*通讯作者。